Surface Photocatalysis and Chemoselectivity in Heterogeneous Catalysis
1) Surface Photocatalysis
Aim and Motivation
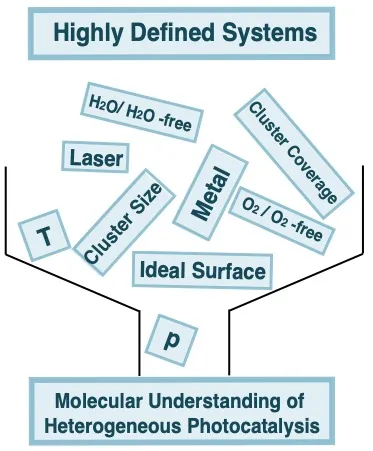
One of the biggest challenges of modern science and technology is the utilization of sunlight for a sustainable energy production. While sunlight already represents the main energy source for chemical processes in nature, human technology hardly makes use of light energy. This may be due to inefficiencies of current systems, which cannot compete economically with existing applications. A reason for this may be a lack of understanding in the fundamental processes involved. In this regard, fundamental scientific work in this area is needed to finally obtain a molecular-level understanding of photocatalysis. In our research, we apply highly defined systems under highly defined conditions in order to achieve this goal. In photochemistry, chemical reactions are driven by light. This opens up new reaction pathways, which are often not accessible by conventional methods in organic and inorganic chemistry. Certain “holy grail” reactions in chemistry, such as water splitting and CO2 activation, may become applicable by this approach. For the technical feasibility, it is inevitable that photoreactions can be driven catalytically by the use of a light absorbing material, which is not consumed during the reaction. In the majority of systems, this so-called photocatalyst is represented by a (modified) solid semiconductor. As the reactants are either in gas or liquid phase, such systems are generalized under the term “heterogeneous photocatalysis”.
Our Scientific Approach
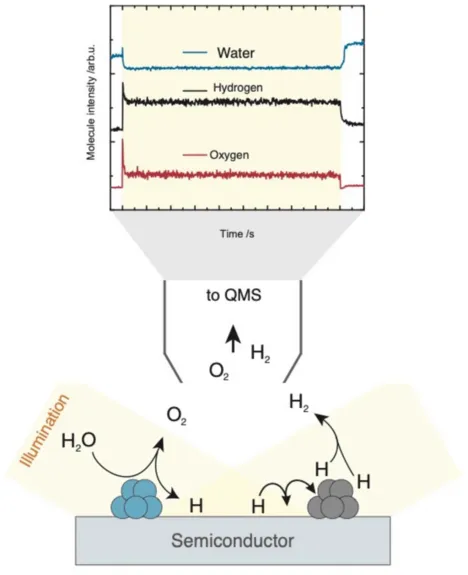
We apply highly defined semiconductor materials (e.g. single crystals), which are either decorated with atomically precise metal(oxide) clusters or studied directly. In heterogeneous photocatalytic systems, the light-driven chemical reaction takes place on the surface, which is covered with the reactant (also called “substrate”). The photoreactions are performed in an ideal reaction environment (i.e. defined pressure and the absence of impurities) and with lasers as light sources. This approach allows to derive generally valid principles and mechanisms by varying certain parameters judiciously (e.g. cluster size and coverage, the reaction conditions or the composition of the semiconductor). The concepts are further studied in order to access their implication to systems working under ambient conditions (e.g. in solution) and relevant in the production of sustainable energy.
Photocatalytic Reaction Mechanisms

In a semiconductor, the electron-filled valence band and the empty conduction band are separated by an energy barrier, the so-called band gap. Upon irradiation of the semiconductor (the catalyst) with light of sufficient energy, an electron is excited into the conduction band. In this process, a positively charged hole (h+) remains in the valence band due to charge conservation. The electron as well as the hole may now diffuse to the surface, where the substrate is adsorbed. While the acceptance of (photo)electrons lead to the reduction of the substrate molecules, the reaction with (photo)holes lead to their oxidation. Therefore, most studies use a mechanism, in which the reaction are viewed analogously to electrochemical systems. However, we have recently discovered that the evolution of H2 in alcohol photoreforming on platinum-loaded TiO2 rather occurs in a manner similar to heterogeneous catalysis. While the alcohol is oxidized in a photocatalytic site on the semiconductor, the platinum clusters (also called the “co-catalysts”) enable a thermal hydrogen recombination reaction yielding H2. This example highlights the importance of our studies for the elucidation of reaction mechanisms in photocatalysis.
The Setup
Our photochemical studies are carried out under ideal conditions, which is in ultra-high vacuum. The setup enables the preparation of clean and defined semiconductor materials as well as their chemical analysis. Furthermore, a laser vaporization cluster source enable the decoration of the photocatalyst with clusters of an atomically defined size and precise chemical composition with freely selectable coverages. The photoreactions are studied by illuminating the as-prepared photocatalysts and their exposure to a reaction environment. Lasers are used as illumination source, which are available in a spectral range from 210 nm to over 800 nm. The experiments are performed at isothermal conditions. While a large temperature range (from 100 K to over 1000 K) is accessible, the reactions are usually conducted around room temperature or below. The photocatalytic reaction is evaluated by the analysis of the reaction products and the efficiency of their formation.
2) Chemoselectivity in Heterogeneous Catalysis
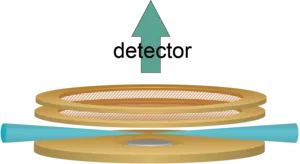
Selectivity of catalytic reaction is of paramount importance, in particular for potential industrial applications. For example, the chemoselectivity of hydrogenation reaction [B1, B2] is crucial for the production of fine chemicals and is still subject to strong research efforts. For many reactions isomers or other products with an equal in mass are formed. Prominent example are the ethylene oxidation to either ethylene oxide or CO2 or, the hydrogenation of unsaturated ketones (or aldehydes), where either the keto group or the double bond of the molecule is hydrogenated. A powerful method, which is very often applied to analyze catalytic reactions under very well defined conditions in the ultra-high vacuum, is electron impact ionization (EI) with a subsequent detection in a quadrupole mass spectrometer (Q-MS). However, the discrimination between products of the same molecular weight is often very cumbersome or even deteriorated because of the unselective ionization technique. To overcome this problem we have developed time-of-flight mass spectrometer (ToF-MS), which enables ionization with lasers. While the ToF-MS enable the simultaneous detection of all masses, the laser ionization is a soft and selective ionization technique. By an excitation in resonance with an electronic state of the analyte molecules, discrimination by the spectral properties is additionally achieved. This resonance enhanced multiphoton ionization (REMPI) can be performed with high sensitivity and is used in temperature-programmed reaction (TPR) spectra in our group[B3]. Finally, TPR in combination with the REMPI-ToF-MS allows for the study of the chemical selectivity in heterogeneous catalytic reaction via the formation of particular isomers at certain temperatures.
References
[A1] | J. Kiermaier, PhD Thesis, 2013, Technische Universität München. |
[A2] | M.A. Henderson, “A surface science perspective on TiO2 photocatalysis”, Surf. Sci. Rep., 2011, 66, 185. |
[A3] | U. Diebold, “The surface science of titanium dioxide”, Surf. Sci. Rep., 2003, 48, 53. |
[A4] | Idriss et al., “The effect of gold loading and particle size on photocatalytic hydrogen production from ethanol of Au/TiO2 nanoparticles”, Nat Chem, 2011, 3, 489. |
[A5] | Walenta et al., "Ethanol Photocatalysis on rutile TiO2(110): the role of defects and water", Phys. Chem. Chem. Phys., 2015, 17, 35. |
[B1] | Witte et al., “BASF NanoSelect™ Technology: Innovative Supported Pd- and Pt-based Catalysts for Selective Hydrogenation Reactions”, Top. Catal., 55, 505. |
[B2] | Davis et al., “Mechanistics Insights on the Hydrogenation of α,β-Unsaturated Ketones and Aldehydes to Unsaturated Alcohols over Metal Catalysts”, ACS Catal., 2, 671 |
[B3] | Winbauer et al., "Isomer-Selective Detection of Aromatic Molecules in Temperature-Programmed Desorption for Model Catalysis", Anal. Chem., 2016, 88, 5392 |
Collaborations
- Dr. C. Henry (Director CINAM-CNRS, Luminy)
- Prof. M. Stutzmann (Experimental Semiconductor Physics and WSI, TUM, Munich)
- Prof. C. Friend (Harvard University, Cambridge)
Project Funding
- Deutsche Forschungsgemeinschaft (DFG)
- Deutsch-Französische Hochschule (DFH)
- European Research Council (ERC) - Advanced Grant
- Nanosystems Initiative München (NIM)